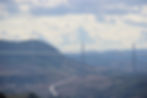
Context:
The Millau Viaduct is a multi-span cable-stayed bridge built from 2001 - 2004, and is not only the most famous bridge in France, but one of the most famous bridges in the world. It was conceived by structural engineer Michel Virlogeux, who was also one of the chief engineers in the Normandy, Jacques Chaban-Delmas and Île de Ré bridges, and was designed by British architect Norman Foster. The bridge carries the A75 motorway over the Tarn valley between Causse Rouge in the north and Causse de Larzac in the south, in order to decongest the nearby town of Millau, through which much traffic would pass in the holiday season due to there being no alternative way to cross the Tarn valley in order to reach the south of France.
The bridge was considered amongst many other propositions, however the design was selected due not only to its aesthetic nature, but also due to its relative proximity to the town of Millau, whose inhabitants were worried that whilst the construction of an alternative route to the south would increase efficiency of travel, they would lose much business from passing holiday-makers. Whilst the bridge is a great technical feat, it is marvelled at by many due to its beauty. From a distance, the cables supporting the deck disappear in the horizon, and the piers and pylons appear as though needles protruding from the ground, a nod by the architect Norman Foster, to the regions’ historical glove-making industry.
Structure:
The bridge is 2460m in length with a radius of curvature of 20,000m. It is composed of eight cable-stayed spans, two end spans with a length of 204m each and 6 central spans of 342m each. The bridge has an incline of 3.025% from from Béziers in the North, to Clermont-Ferrand in the south, and has seven concrete piers, the tallest of which, together with the pylon atop it, has a height of 343m, 19m taller than the Eiffel Tower, and the smallest of which is taller than the Abbey of the Mont St. Michel, thus earning the Millau Viaduct the title of the tallest bridge in the world. The top 90m of each pier is split into two shafts, each of which is made using prestressed concrete.
The deck is a steel trapezoidal box girder with a maximum height of 4.2m, an overall width of 32m, and a dual carriageway width of 23.8m. The upper deck is orthotropic consisting of longitudinal ribs resting on girder crossing elements. Two vertical webs of between 20 - 40mm thick in the centre of the trapezoidal box girder run the length of the deck.
The pylons, are composed of a split shaft of 38m in length, each shaft of which is composed of a steel girder, and a 49m mast onto which the cables are anchored with 17m of additional steel at the top of each pylon for aesthetic purposes. The split shaft of the pylons and the piers are orientated in the same direction, longitudinally for both structural and aesthetic purposes.
There are 11 pairs of cables anchored to the central reservation of the deck in a half-fan longitudinal arrangement. These cables consist of between 45 and 91 T15 strands of steel having a Young’s Modulus of 1,860MPa and are super-galvanised, sheathed and waxed. There is also an aerodynamic sheath around each cable. The steel used in the deck and pylons is of grade S355 and S460, whilst the concrete used in the piers is of grade B60.
Structural Behaviour:
The Millau Viaduct is a multi-span cable-stayed bridge. This means that it is a cable-stayed bridge which has more than 3 spans. A cable stayed bridge is one in which cables are anchored to pylons fixed to the bridge, and to the bridge deck. The cable-stays are in tension and transfer the load of the deck to the pylons and piers which are in compression. The benefits of cable-stayed bridges are that the cable-stays are very efficient at carrying loads, and the risk of the deck buckling is relatively small, meaning that it is possible to construct bridges with very long spans. However there are significant difficulties in designing a multi-span cable-stayed bridge in that the effects of wind, temperature, dead and live loads will all be more complex, given that where one of the above factors affects one span, all other spans will also be affected. The 14 years which the Eiffage group responsible for the construction of the Millau Viaduct spent researching the bridge’s structural behaviour before the beginning of construction are testament to this difficulty.

In a single main cable-stayed span, the cable-stays are anchored at the abutments, ensuring that there is no movement in the pylons. This is not the case in the Millau Viaduct given that of the 8 spans only two have cable-stays anchored at abutments. When a span is loaded, the pylon will be deflected towards the span which is not loaded. This is taken into account in the bridge’s design by fixing the deck to both the piers and the pylons, reducing the vertical movement in loaded spans, to decrease pylon deflection. By increasing the rigidity of the piers and pylons, it became possible to decrease the thickness and load of the deck, resulting in the thin steel trapezoidal girder being used as the deck.
This has further benefits to the overall design due to the high winds, caused by an increased tunnelling factor as a result of the bridge’s location in the valley, as along with its trapezoidal shape, the decreased thickness of the deck means that the deck is more aerodynamic and less susceptible to lifting, which is a common issue amongst suspension and cable-stayed bridges. Whilst, the fixing of the deck to the piers and pylons reduces the knock-on effects of load and the risk of the deck lifting, this also creates difficulty when considering how best to design the bridge to endure thermal dilation and the creep and shrinkage of concrete.

The calculated maximum possible displacement of the piers at each end of the bridge, due to a thermal dilation of 40ºC is 0.6m. However the rigidity of the piers caused by the fixation of the deck to both the piers and the pylons means that in reality, were the end piers to be displaced by 0.6m they would not be able to resist the forces placed upon them. The solution to this problem was to split each of the end piers into two shafts for the last 90m of each of the piers, which ensures that, by doubling the amount of bearings in the longitudinal direction atop each of the piers - two on each shaft of the pier - the structure’s bending was reduced. Whilst the split pier was only necessary for the end piers, which are subject to the greatest bending forces in the thermal dilation of the deck, for aesthetic purposes the split shaft pier and pylons were adopted throughout the bridge.
Concerning the trapezoidal girder deck, the two vertical webs that run through the entire length of the deck were put in place to redistribute the force from the temporary piers during the deck launching. The orthotropic decks - longitudinally ribbed steel sheets placed on cross-girder elements - are a lightweight method of providing strength under compression to the upper surface of the deck.
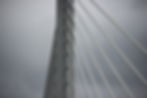
Another important aspect of the design is the sealing and aerodynamics of the cable-stays. The cable-stays are super galvanised, sheathed and waxed, all of which reduce the risk of corrosion by decreasing the likelihood that they come in contact with air. In addition, each cable-stay is surrounded by a white sheath, textured with discontinuous circular patterns in order to disrupt airflow, reducing the risk of the cable-stays vibrating. If any of the cable-stays were to vibrate significantly, the impacts on the entirety of the structure would be large, including possible bending of the piers and pylons due to a loss of tension in the cable-stays. Moreover the way in which these cable-stays are arranged, in a half-fan means that the axial force in the girder is smaller than that of radial or harp longitudinal arrangements.
Construction:
The construction of the Millau Viaduct was significant in how it lead to the development of a new hydraulic launching system, designed by Eiffage, the engineering group leading the build. Due to the enormity of the project, each pier - denoted P1 to P7 - was considered to be an individual building site. Each pier was built above four foundation wells, in which a cylindrical steel frame has been filled with concrete. These were each 5m in diameter and 9 to 18m in depth, in order to support the large dead and live loads of the bridge. The hollow piers were added to at the rate of four metres a day, using a self-climbing formwork for the outside and crane-assisted formwork on the inside. The formwork was adjustable to accommodate the changing shape of the piers over their height. Due to the height of some of the piers, the tallest being - P2 at 245m - cranes were used to pump the concrete into the frame.
A key element to the construction phase of the development was the erection of the temporary piers. These were 7 steel K-trussed piers erected halfway between each of the concrete piers, in order to support the deck in the launching phase. At the top of these temporary piers a trimmer was fitted in order to hold the hydraulic launching systems, called translators.
These translators were essential to the launching of the steel deck, which was assembled on site from parts transported from factories in Lauterbourg and Fos-sur-Mer. The assembly of each 171m of deck took approximately four to five weeks with the participation of 75 welders, working full time. The launching of the deck was very difficult due to the extent of accuracy which it required. The deck launching began in July 2003 from the south end where 1,743m of deck needed to be constructed and a year later, began from the north end, where only 717m of deck needed to be constructed. The two decks which were launched first on either side of the bridge met in between pylons 2 and 3 and were the only decks to have their pylons attached as well as the cable-stays which had already been anchored to the deck and partially tensioned. This was in order to lend some support to the decks as they were sent out perilously into the void.
The equilibrium devices and translators - used to launch the deck - totalling four of each, atop each of the temporary and concrete piers, and were all centrally controlled to ensure that the movement of the deck was uniform and well calculated. The translators are each composed of a U-shaped cradle under which a lifting wedge is slowly pushed by the lifting jacks.

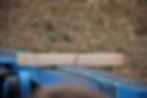
The translators work in the following manner : In the first instance the deck is supported by the cradle. The lifting jack, able to lift 250 tonnes, then makes the lifting wedge move back under the cradle, lifting it from its original resting position. With the cradle raised from its supports, the rails under the cradle move it forward with pressure from another launching jack and then the wedge returns to its first position, leaving the deck resting on the cradle but 0.6m further along.
With this machinery, which is now employed in countless bridge constructions worldwide, the deck was launched at the rate of 10m per hour, enabling steady progress. The cable-stays anchored to the leading decks during launching were only partially tensioned. This decreased their vibration frequency making them more susceptible to vibrating in wind. To counter this, parallel cables were attached between the cable-stays to increase their vibration frequency. Once the decks had been launched, there was constant meteorological surveillance, and the launching was made to stop immediately if wind gusts of greater than 85km/h were detected due to the threat this posed to the relatively light deck, which was not yet fixed to the piers nor pylons.
The the launching systems proved to be infallible and the two decks met in June 2004. After the laying of the road, the remaining pylons were fitted and the cable-stays were anchored and tensioned. The deck, which due to its flexibility had an undulating profile upon launching, became straight after the tensioning of all the cable-stays, and the construction was complete.